A Rare Case of Hereditary Fructose Intolerance
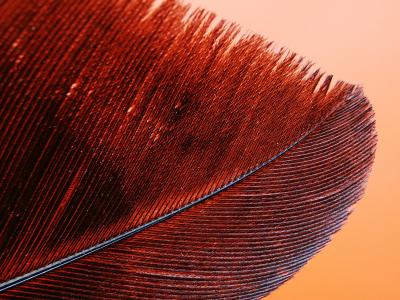
Abstract
Hereditary fructose intolerance is a very rare autosomal recessive metabolic disorder, with unknown global prevalence. Carrier frequency is estimated at approximately 1 in 70 individuals, especially in those of Caucasian origin. The key enzyme in fructose metabolism is aldolase B produced by the liver at low constant levels. Due to mutations in the ALDOB gene (located on chromosome 9q31.1), the enzyme activity can be drastically reduced (85-100%). In Europe, more than 80% of mutations are p.A150P, p.A175D and p.N335K. We present a case of a 15-month old patient with a history of liver insufficiency, who was admitted for failure to thrive, fever and diarrhea. During admission, acidosis, coagulopathy, hepatomegaly and coma added to the clinical picture, among other symptoms. The results of clinical and paraclinical evaluations raised the question of hereditary fructose intolerance vs. hydroxyglutaric aciduria.
Genetic testing revealed that the patient was a compound heterozygote having the p.A174D (c.524C>A) mutation in exon 5 and a 4 bp deletion in exon 3 (c.113-1_115delGGTA) of ALDOB gene. Subsequent genetic testing of the parents showed that they were both heterozygotes: the father for the p.A174D (c.524C>A) mutation and the mother for the 4 bp deletion in exon 3. The particularities of this case are the presence of liver insufficiency, acidosis and coma in a child, without ketonuria and neurological long-term consequences, that was eventually diagnosed as hereditary fructose intolerance.
Table of Content:
1. Background
2. Case Report
3. Discussions and Conclusion
4. Disclosure
1. Background
Hereditary fructose intolerance (HFI), also known as fructosemia (OMIM #229600), is a rare autosomal recessive disorder of metabolism resulting from a deficiency in aldolase B activity, which is the main enzyme involved in hepatic metabolism of dietary fructose. As a result, the body is unable to metabolize correctly sugars such as fructose, sucrose or sorbitol which leads to toxicity and possible severe organ damage or even death in case it remains undiagnosed and untreated [1], [2].
HFI incidence in European countries is reported to be between 1:30,000 and 1:20,000 in Caucasian individuals [1], [2], with a carrier frequency of 1:70 to 1:50 [3], [4]. In the United States, the incidence is estimated at 1:60,000 individuals (ranging from 1:38,000 for Middle European individuals to 1:205,000 for African-Americans) [5], and the carrier frequency at 1:122 individuals [5].
In normal situations, dietary fructose is absorbed from the small intestine with the help of GLUT5 (a specialized transporter located in the apical cellular membrane) and GLUT2 (a hexose transporter which is located in the basal cellular membrane). After absorption, fructose is metabolized in the liver by hexokinase IV (glucokinase) and fructokinase (FK), the latter being the main metabolic pathway, to fructose 6 phosphate (F6-P) and fructose 1 phosphate (F1-P), respectively. F1-P enters the downstream catabolic (glycolysis) or anabolic (gluconeogenesis) pathways after cleavage to glyceraldehyde and dihydroxyacetone (DHAP) phosphate due to aldolase B. Deficiency of aldolase B activity leads to a reduced cleavage of F1-P that triggers a toxic complex biochemical cascade with consequences beyond the liver, including the presence of high blood and urine levels of fructose [1]. Among the toxic effects there are the formation of intracellular precipitates in the liver and kidney producing cellular necrosis and organ failure in severe cases, severe hypoglycaemia, phosphate depletion that further triggers hypermagnesemia, hyperuricemia and metabolic acidosis, hypophosphatemia, hyperalaninemia [1] or even death [3].
The clinical phenotype usually manifests in young children after sucrose- and fructose- containing foods are introduced in the diet, with bloating, abdominal pain, nausea and vomiting [1], [2] and liver and kidney damage long-term effects, in case it remains undiagnosed and untreated [1]. Children develop an aversion for sweets and fruits, which is also associated with an absence of dental caries that together with the aforementioned symptoms can lead to a suspicion of HFI [2].
Diagnosis is sometimes difficult since symptoms are similar to many other disorders, including metabolic diseases, such as FK-1 deficiency (or essential fructosuria), in which fructosemia and fructosuria also appear after fructose ingestion, intestinal GLUT5 transporter deficiency, in which symptoms like abdominal pain and nausea also occur, or type 1 galactosemia, where liver and kidney damage is a common complication [1].
In previous years, positive diagnosis of HFI was possible through an intravenous fructose load test or an enzymatic assay on liver, intestinal or kidney biopsy samples, that both proved to be dangerous for the patient or too invasive [2], [4]. Recently, breath tests and molecular genetics have provided the necessary tools for non-invasive diagnosis of both patients and carriers [2] and the differential diagnosis with other metabolic disorders or fructose malabsorption [6].
Currently the only possible cure for HFI is a strict dietary restriction of fructose and equivalent sugars [1], [2], [4]. Unfortunately, complete elimination of these sugars from a long- term diet is impossible so, despite prompt diagnosis and change in diet, patient might still suffer from toxic effects due to chronic ingestion [1], [5]. Beside the dietary exogenous sources, fructose is also synthesized endogenously from sorbitol due to the hepatic sorbitol dehydrogenase enzyme [1], [6], so this has to be considered when dietary therapeutic measures are introduced [1], [5].
In view of this, research is needed in order to, not only, further improve diagnosis, but to find a way to correct the metabolic deficiency [1]. Gene therapy might be considered as a possible cure for ALDOB gene mutations or administration of FK inhibitors for the prevention of F1-P synthesis and accumulation, and hence of toxic effects [1].
Aldolase B (E.C. 4.1.2.13) belongs to the fructose 1,6-biphosphate (F1,6-P) aldolase enzyme family, together with aldolase A (an enzyme expressed in vertebrate tissues) and aldolase C (an enzyme expressed mostly in the brain) [1]. These enzymes normally participate in the cleavage of F1,6-P, but aldolase B also has similar affinity for F1-P. The major site for aldolase B function is the liver, but it can also be found in intestinal or kidney cells [1].
The encoding ALDOB gene is located on chromosome 9q31.1 and contains 9 exons (spanning over 14.5 kbp) out of which all are expressed but the first one [3] and encodes a 364 amino acid-long protein [1], [2]. More than 40 (some authors report more than 50) gene mutations have been described in the literature, mostly being point mutations that result in a single amino acid change [1], [2], [3], [7] which usually causes the decrease in stability or catalytic activity of the enzyme [1], [2], leading to 85-100% deficient enzyme [1]. Other types of mutations were described: point mutations resulting in nonsense codons or splicing defects, deletions and insertions (ranging from 1 to 1,600 bp) with various effects [3] or intronic mutations [2]. The type and frequency of mutations can vary between ethnic groups and regions [2], three of them accounting for approximately 85% of all mutations in the European population: p.A150P (c.448G>C) – between 61-65%, p.A175D (c.524C>A) – between 11-16% and p.N335K (C.1005C>G) – between 5-8% [2]. The p.A175D variant is reported to be more frequent in the Southern Europe [2], while the p.N335K is found predominantly in patients originating from Southeast Europe [2]. Other mutations are found in specific ethnic groups or families, such as p.A338V (c.1013C>T) [2], [8], p.R60X (c.178C>T) [2], [9], p.N120KfsX30 (c.357delAAAC) [2], [9], p.K108K (c.324G>A) [2] and c.625-1G>A [2]. In the Hispanic and African-American populations the most frequently reported mutations are p.A150P and p.A175D [3], while in Northern India the most frequent mutation was reported to be c.324+1G>A [5], [10], [11], [12]. In Korean population, a study identified the p.V253fsX24 mutation [7] and in Brazilian population, a study reported that p.A150P mutation was less frequent compared to p.A175D, p.N120KfsX30 or p.R60X [13]. In the United States the most frequent mutations were reported to be p.A150P and p.A175D [13].
No genotype-phenotype correlation has been reported for HFI so far [5], the severity of toxic effects being directly correlated with the individual dietary habits [5].
In many cases still, the mutations remain unknown [3], ranging from more than 10% [12] to over 33% of cases in the American population [12] or up to 60% in non-Caucasians such as African-Americans or Hispanics [3].
Genetic analysis has become a very useful and important tool for HFI diagnosis since 2005 [2], replacing the invasive and potentially dangerous methods previously used [2]. Currently, genetic testing includes single-gene analysis (such as sequencing, gene-targeted deletion/duplication analysis and targeted analysis of pathogenic variants) [5], multigene panel (that includes the ALDOB gene among other genes of interest) [5] and comprehensive genomic testing (such as whole-exome – WES, whole mitochondrial – WMitoSeq or whole-genome sequencing – WGS) [5]. Sequencing analysis is the first to be performed in case there is no suspicion of a particular pathogenic variant (i.e. positive family history and/or known ethnicity) [5] and can detect between 66% to 100% of cases; when only one or no pathogenic variant is identified, analysis of gene deletions/duplications can be used (e.g. quantitative PCR, long- range PCR, multiplex ligation-dependant probe amplification – MLPA, gene-targeted microarray) with a rate of detection between 0% and 20% [2], [5], [9], [14], [15].
Still, in some situations, current technology is not able to identify any mutations, which suggests that new investigations need to be introduced in clinical practice, such as RNA analysis, promoter or intron sequencing [2].
2. Case Report
We present the case of a 1.3 year-old old boy who was admitted to Pediatrics Hospital for fever (38.2 °C), lack of appetite, failure to thrive, and diarrhea. The personal history revealed an episode of liver failure (4 months prior to current admission) that was diagnosed as acetaminophen intoxication, no weight gain (since 3 months ago), and episodes of diarrhea (since 1 month ago).
On admission, the physical examination showed pallor, low weight, diminished subcutaneous tissue, and signs of rickets, despite a relatively good general status. The paraclinical examination revealed absence of inflammatory markers, in the presence of hepatic cytolysis markers, positive Epstein-Barr virus (EBV) IgM antibodies, and positive stool analysis (Klebsiella oxytoca and enteropathogenic Escherichia coli). Probiotics, antipyretics, trimethoprim/sulphamethoxazole and lactose-free milk formula were introduced as treatment.
Under therapy, the general status became worse with persistence of fever, diarrhea, vomiting, increasing inflammatory and hepatocytolysis markers. Cholestasis syndrome, coagulopathy and uncompensated non-lactic acidosis added to the clinical picture so treatment with intravenous arginine and immunoglobulins, oral ursodesoxycholic acid and intravenous rehydration solutions were administered.
The patient was transferred to the ICU, with the diagnosis of severe acute hepatitis, recurrent liver insufficiency, acute EBV infection and enterocolitis. Blood tests revealed high levels of liver transaminases (ASAT 1,779 U/L; ALAT 858 U/L) and lactate dehydrogenase (LDH) (1,279 U/L), increased alkaline phosphatase (AP) (534 U/l), γ-glutamyl transferase (γGT) (102 U/L), total bilirubin (4.77 mg/dL) and direct bilirubin (3.91 mg/dL). Signs of dehydration persisted; hepatomegaly (6 cm below the costal margin) and axial spasticity were also observed.
In evolution, the patient became comatose (Glasgow score 8) with severe hypoglycemia, non-lactic acidosis, but without ketonuria.
Considering this, coma of metabolic origin was suspected so the following tests were performed: ammoniemia (34 μMol/L), plasma amino acids (within normal range), urinary amino acids (increased Ser, Thr, Gln, Ala), urine organic acids analysis (that was available later), acylcarnitine profile (within normal range), serum lactate-to-pyruvate molar ratio (within normal range). Treatment with intravenous glucose 20%, oral glucose and lipid solutions, dietary protein restriction was introduced leading to an improved general status, normal stools, normalization of liver enzymes and of coagulation tests, still with lack of appetite and weight gain.
After a two-week period, urine organic acids analysis revealed increased 2-oxoglutaric and hydroxylutaric acids, raising the question of HFI vs. hydroxyglutaric aciduria. Both diseases have similar clinical and laboratory findings, with craniofacial dysmorphism (micro- /macrocephaly), encephalopathy, and variable hyperammoniemia being more characteristic for hydroxyglutaric aciduria, while malnutrition, vomiting, liver failure, coagulopathy, coma, and ketonuria for HFI.
Genetic Testing
DNA isolation was performed on fresh whole blood samples obtained from the patient, using the Quick-gDNATM Blood MicroPrep kit (ZymoResearch, USA) and protocol. Subsequent DNA testing was performed using the Sugar Intolerance StripAssay (ViennaLab Diagnostics, Austria) reagents and protocol that is able to identify four frequent mutations. The genotype was determined using the CollectorTM sheet enclosed in the kit as being heterozygote for the missense mutation p.A174D (c.524C>A; p.Ala175Asp) in exon 5 of the ALDOB gene.
DNA sequencing was performed on 8 exons and flanking intronic sequences (NM_000035)
of ALDOB gene and revealed a 4 bp deletion (c.113-1_115delGGTA) in the acceptor splicing site of exon 3, which is listed as a pathogenic mutation in the ClinVar database (RCV000169352.1).
Genetic Diagnosis
Subsequently, the diagnosis was of HFI by compound heterozygosity, with the ALDOB NM_000035: c.[113-1_115del]; [524C>A] genotype. Gene sequencing of exons 3 and 5 in the parents showed that they were both heterozygotes: the father with the p.A174D (c.524C>A) mutation in exon 5 and the mother with the 4 bp (c.113-1_115delGGTA) deletion in exon 3, which confirmed that the pathogenic recessive alleles found in our patient were inherited from the parents.
Evolution
Due to positive diagnosis of HFI, strict dietary restriction of fructose was recommended, associated with restriction from other similar sugars, avoidance of long fasting periods, Pneumococcal and Haemophilus influenzae vaccinations. The general status of patient became normal, with normal neurologic and cognitive development, weight gain, normal blood and urine analysis and normal hepatic ultrasound.
3. Discussions and Conclusion
HFI is a rare autosomal recessive metabolic disease caused by a deficiency in aldolase B enzyme that leads to toxic effects that frequently are misdiagnosed because the symptoms and laboratory findings overlap with many other metabolic disturbances [1], [2], [16]. In some cases, symptoms might actually be caused by acquired fructose intolerance due to the damage of the small intestine epithelial cells that leads to fructose malabsorption [6], [16] or dietary protein-induced enterocolitis [17]. Usually, fructose malabsorption can be diagnosed by a fructose load test (H2 breath test), but only after HFI is ruled out using genetic testing because of the possible life-threatening symptoms that the load test might provoke in individuals with HFI [6].
Patients with delayed or no diagnosis will survive in most cases because they develop an aversion for fructose-containing foods [16], but in some situations life span can be reduced because of the severity of liver and kidney damage, or liver cirrhosis (fibrosis) will gradually appear since complete elimination of fructose from the diet is impossible [16]. Differential diagnosis of fructose malabsorption is also crucial for a normal life span, since treatment in this case would involve a fructose-modified diet, with increased lipid and protein content that is stimulating the absorption of fructose from the digestive tract [6].
The molecular genetic analysis of ALDOB gene has become the most important diagnostic tool today for HFI, showing an increased frequency of several mutations across countries and ethnic groups [13]. In Romania, the prevalence of HFI and of the causing mutations still remains unknown, although a recent study reported a case with the p.A175D homozygote mutation [16] that is similar to our findings, and suggests that this is probably the most frequent pathogenic variant in Romanian population (with Caucasian origin). The other mutation in our patient and in one of the parents, namely c.113-1_115delGGTA, is listed as pathogenic in the ClinVar database, but, to our knowledge, there are no other reported cases in the literature except the case noted by Cross and Cox [17].
Usually HFI symptoms do not appear during breast-feeding, and sometimes are triggered by a metabolic decompensation due to drug administration for infections [18]. In our case, the symptoms appeared after an episode of liver insufficiency triggered by previous administration of trimethoprim/sulphamethoxazole. Gradually, in our patient clinical and laboratory findings became suggestive for HFI, after hydroxyglutaric aciduria was ruled out. Genetic testing confirmed the suspicion, and immediate removal of fructose from diet normalized the phenotype of patient. The particularities of this case are the presence of liver insufficiency and coma in a child, but without ketonuria and neurological long-term consequences which are usually seen in HFI. To our knowledge, despite the continuing fructose-free diet, the patient has recently developed signs of liver fibrosis, without any other complications.
In conclusion, we draw attention to the fact that HFI might be considered as a diagnosis in children and adults when certain symptoms and signs appear, even if in some cases the phenotype is not complete; genetic testing is the only safe and reliable test that would confirm the diagnosis.
4. Disclosure
The authors have no conflicts of interests to declare.
Informed and written consent was obtained from the patient’s parents prior to DNA isolation and genetic testing.
The Authors:
MILITARU Mariela Sanda [1][2]
MILITARU Mihai [3]
MARIȘ Alexandra [3]
ȘTEFĂNUȚ Maria [2]
POP Mihaela [2]
AIBEN Iulia [2]
MILITARU Diana [1]
CĂTANĂ Andreea [1]
GUG Cristina [4]
JURCĂ Claudia [5]
BRAHA Elena [6]
DRONCA Eleonora [1]
[1] Iuliu Hațieganu University of Medicine and Pharmacy, Cluj-Napoca (ROMANIA)
[2] Genetic Center, Cluj-Napoca (ROMANIA)
[3] 2nd Paediatrics Hospital, Cluj-Napoca (ROMANIA)
[4] Victor Babeș University of Medicine and Pharmacy, Timișoara (ROMANIA)
[5] University of Medicine and Pharmacy, Oradea (ROMANIA)
[6] National Institute of Endocrinology “CI Parhon” Bucuresti (ROMANIA)
Contributo selezionato da Filodiritto tra quelli pubblicati nei Proceedings “5th Medical Genetics Congress with International Participation - 2018”
Per acquistare i Proceedings clicca qui.
Contribution selected by Filodiritto among those published in the Proceedings “5th Medical Genetics Congress with International Participation - 2018”
To buy the Proceedings click here.