Blockchain, cryptocurrencies and the environmental question
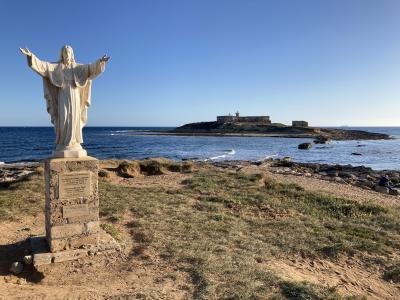
Abstract
Crypto-currencies have been criticized for having a large carbon footprint. But is this criticism grounded? And if that is the case, what are the alternatives? This short primer aims to analyse the premises of this debate, present the current studies conducted on this subject and provide an outlook of the most discussed conclusions drawn by experts.
1. The environmental question
“Tesla has suspended vehicle purchases using Bitcoin. We are concerned about rapidly increasing use of fossil fuels for Bitcoin mining and transactions, especially coal, which has the worst emissions of any fuel. Cryptocurrency is a good idea on many levels and we believe it has a promising future, but this cannot come at great cost to the environment. Tesla will not be selling any Bitcoin and we intend to use it for transactions as soon as mining transitions to more sustainable energy. We are also looking at other cryptocurrencies that use <1% of Bitcoin’s energy/transaction” (Musk, 2021).
Thus tweeted Elon Musk, CEO of Tesla, just two months ago, causing an immediate plunge of BTC and the cryptocurrency market in general. Unsurprisingly, the tweet reignited an already heated debate on the cryptocurrencies’ environmental credentials, often polarised between those who believe that blockchain applications are capable of counterbalancing their carbon footprint and those who – on the other hand – question (especially in the short run) the soundness of such theses.
As this brief primer will show, both parties have valid arguments to support their stance; a final opinion, therefore, cannot be drawn. Notwithstanding this, one will notice that both sides still agree – to a certain extent – on a common ground, which can thus provide for a useful starting point to this analysis: firstly, the need to address the several misconceptions this topic is fraught with; secondly, the common-sense-based understanding that today such environmental issues represent a critical policy area and therefore should not be discarded, nor overemphasised, light-heartedly.
As for the first point, we can identify a core of five main misconceptions which should be immediately clarified: i) energy consumption is not equivalent to carbon emissions, as different energy sources have a different environmental impact (Hammon et al., 2021; de Vries, 2019); ii) it is currently impossible to determine, with a degree of certainty, the precise “energy mix” – i.e. the energy sources employed – behind the mining of any cryptocurrency (de Vries, 2021a; Carter, 2021a, 2021b; Gallersdörfer et al., 2020; Blandin et al., 2020); iii) the mining process of a cryptocurrency should be distinguished from the actual transactions involving that cryptocurrency, which per se have in most cases a negligible environmental impact (Carter, 2021a); iv) besides the mining process, there are several ancillary activities – such as cooling, off-chain transactions, and e-waste management – that should be taken into account in the assessment (de Vries, 2021b, 2019; Blandin et al., 2020); v) an environmental impact assessment of cryptocurrencies solely based on the study of Bitcoin will fall short of its premises, as the latter – albeit being by far the most prominent cryptocurrency – is still not responsible for the total energetic consumption and, at the same time, does not fairly benchmark other existing blockchain systems which have much lower carbon footprints (Hammon et al., 2021; Smith, 2021; Blandin et al., 2020; Gallersdörfer et al., 2020; de Vries, 2019; Stoll et al., 2019).
As for the second point, we should bear in mind that in the end – as recently stressed in an article of the Harvard Business Review – “figuring out how much consumption is too much is a complex question that’s intertwined with debates around our priorities as a society. The calculation of which goods and services are worth spending these resources on, after all, is really a question of values.” (Carter, 2021a).
In the following chapters, an outline of several studies’ main outcomes on Bitcoin’s environmental impact is presented (§2), taking into consideration the five misconceptions aforementioned. Subsequently this primer will report the current studies – inside and outside of the Bitcoin’s blockchain system – conducted to address the environmental concerns and find viable solutions (§3).
2. Case study: Bitcoin (and beyond)
Bitcoin is today the most prominent cryptocurrency in the world: as of July 19 2021, its market capitalisation amounted to 586.4 billion USD, after reaching an impressive peak of 1100 billion USD just in the past few months. Bearing in mind that these figures cannot replace a comprehensive assessment of other cryptocurrencies too (see §1, misconception (v)), there are at least three reasons why this research should nonetheless start from Bitcoin: firstly, as shortly explained hereunder, Bitcoin is considered the first cryptocurrency (or better, the first successful attempt to validate transactions via a decentralised data protocol) and hence the driver of the cryptomarket upsurge in the past seven years; secondly, many cryptocurrencies today are still based upon Bitcoin’s blockchain system; thirdly, given its importance, Bitcoin has been object of much more thorough and validated studies.
Bitcoin was introduced in 2008 by its pseudonymous founder Satoshi Nakamoto. The founding idea was to create a “purely peer-to-peer version of electronic cash” (Nakamoto, 2008), which would have allowed “online payments to be sent directly from one party to another without going through a financial institution”. As Stoll et al. (2019) pointed out, “[t]he revolutionary element of Bitcoin was not the idea of a digital currency in itself” but, rather, its “underlying technology”. This technology, indeed, created for the first time the possibility for participants to validate transactions and ensure the integrity of the network without a central trusted party (e.g. a bank). We call this technology “blockchain”, and the one created by Satoshi has since been referred to as the “first blockchain” (Yaga et al., as cited in Stoll et al., 2019, p. 1647). Unfortunately, blockchain – or better, the protocol which makes it function, called “distributed ledger protocol” – constitutes the first and most important hindrance behind the environmental question. A deeper look into the technical facets of this problem seems therefore opportune.
In short, the blockchain is a distributed ledger in which (blocks of) transactions are recorded. In order to create these blocks and for make them transparent and tamper-proof (the overall activity is called “mining process”), hence usable for decentralised financial transactions, Satoshi’s protocol – and, as stated above, many other cryptocurrencies as well – use an algorithm, called “Proof of Work” (PoW), which requires specialised hardware and vast amounts of electricity. As Hammon et al. (2021) put it, “Proof of Work involves solving a mathematical puzzle […]. The puzzle is not conceptually complex, but is difficult in the same way that, say, finding the four numbers to open a combination lock is difficult (if you start at 0000 and work up to 9999, at some point the lock will open)”. Similarly, miners race to solve the mathematical puzzle, and the lucky miner who guesses the number first gets rewarded with a certain amount of new Bitcoins (Smith, 2021)[1].
The electricity needed to run the mining hardware for Proof of Work calculations is what generates the huge energy demand of Bitcoin. This issue is amplified by two factors. First, most other major cryptocurrencies use a very similar mining/confirmation process and so generate similarly high energy consumption levels. Second, “the difficulty of these puzzles adjusts regularly, in order to account for changes in connected computing power and to maintain approximately 10 min between the addition of each block” (Narayanan A. et al., as cited in Stoll et al., 2019, p. 1647). This explains why, with the upsurge of cryptocurrencies mined in the market, the difficulty of PoW increased and so did the energetic consumption, which is now often compared to annual consumption of entire countries. This effect is amplified by the fact that “unlike other financial assets, Bitcoin [and all other cryptocurrencies based on the same system]uses more resources as its price goes up” (Smith, 2021): indeed, when the price increases, each lottery becomes more valuable, hence more miners are lured into the race and the electricity consumption grows. As of today, according to Cambridge Center for Alternative Finance (CCAF) latest data, Bitcoin consumes around 130 Terawatt Hours (TWh) per year, and other Indexes report similar values: Digiconomist, for example, indicates an annual consumption of 120 TWh. To make the idea, that amount of energy constitutes roughly 0,55% of the global electricity production, and would be sufficient to entirely power nations like The Netherlands and provide about half of the energy required by Australia (Carter, 2021b; de Vries, 2021a).
On the other hand, as it was pointed out in the first misconception’s caveat, energy consumption is not necessarily an environmental problem per se, whereas it becomes one when it translates into a huge carbon footprint. We, therefore, have to know how the required energy is produced. And in order to accomplish that, since it is impossible to calculate the exact amount, we should know where the energy is coming from. As explained by de Vries (2021a), “[t]he location of miners is a key ingredient to know how dirty or how clean the power is that they are using”, because a more accurate “energy mix” can be inferred. The first results are not promising: “Bitcoin’s biggest problem is perhaps not even its massive energy consumption, but the fact that most mining facilities in Bitcoin’s network are located in regions (primarily in China [latest CCAF’s mining map confirms a country share of ~65% on the overall monthly mining processes]) that rely heavily on coal-based power” (de Vries, 2021a).
Thus, to put it simply, the real problem is that “coal is fueling Bitcoin” (Stoll, as cited in de Vries, 2021a). The problem with that, as is well known, is coal’s massive carbon footprint. In 2019, Stoll et al. (2019) found that the annual global carbon emissions of Bitcoin “range between 22.0 and 22.9 MtCO2”. Compared to the global energy-related CO2 emissions of 30000 MtCO2 in 2016 (International Energy Agency, as cited in Stoll et al., 2019, p. 1654) this might seem small, but it would still rank between numbers 82 and 83 on the list of biggest emitting countries.
On top of that, Bitcoin and similar cryptocurrencies have to address the issue of “environmental impact beyond energy use” (de Vries, 2021b, 2019). The main challenge here concerns the usage and the disposal of mining hardware. The latter is indeed a fundamental variable of the mining equation, since, as explained above, miners constantly run a race to solve the mathematical puzzle and obtain the coin reward. Economically-rational miners, therefore, will choose their hardware on the basis of two factors: performance (the faster the machine computes, the higher the chances to gain profit); and efficiency (the less electricity the machine requires, the less utility costs the miner has to bear). Mining economics have thus led Bitcoin miners, since 2013, to start using application-specific integrated circuits (ASICs)[2] to solve the puzzle. This, however, has drastically reduced the hardware components’ lifespan, without even mentioning the cost increase for other utilities needed, for example, to cool those rigs down. Overall, considering that only the most efficient and performing machines can remain competitive, de Vries (2019, p. 896) calculates that “we can expect mining equipment to become obsolete in roughly 1.5 years” – and what is worse, “for ASIC mining machines there is no purpose beyond the singular task they were created to do, meaning they immediately become electronic waste (e-waste) afterward”.
Finally, going beyond Bitcoin, it is opportune to include estimates for other cryptocurrencies as well. Stoll et al. (2019) suggest that this would add another 30 TWh to their annual energy consumption’s estimate for Bitcoin, but again the real question arising is whether such energy can be obtained in a clean way. Not much research has been yet done on this. What we can observe, however, is that the trend seems to confirm a higher diversification of cryptocurrencies, which could further complicate the assessment: for example, Gallersdörfer et al. (2020) suggest that the cumulative energy demand of alternative crypto-currencies (that is, alternative to BTC) today has become one third of the total. Also, another interesting fact emerges from their analysis: that “ASIC-resistant algorithms consume an overproportionate amount of energy in relation to their market capitalisation”. This means that, quite ironically, the alternative cryptocurrencies’ attempt to reduce e-waste by prohibiting the exploitation of ASICs in favour of all-rounders GPUs might instead cause an uprise in energy demand and thus presumably in carbon footprint, since GPUs are much less energy efficient.
3. Alternatives and viable solutions
Considering all the above, it should be clear that cryptocurrencies are in fact posing an environmental threat. The Author’s personal stance is that running a blockchain system with a (much) milder energetic impact is still possible, with yet some drawbacks, as explored below. The e-waste issue raised, among others, by de Vries, instead, seems likely to remain an unsolved problem in the upcoming years.
The following paragraphs summarize some of the most widely discussed conclusions drawn by experts on the cryptocurrencies’ “environmental question”.
Renewable sources of energy. By far one of the most mentioned alternative, the possibility for miners to compensate for their huge energy demands with renewable energy is certainly appealing. This argument lays on two considerations. First, “almost all of the energy used worldwide must be produced relatively close to its end users – but Bitcoin has no such limitation, enabling miners to utilize power sources that are inaccessible for most other applications” (Carter, 2021a). Secondly, there are areas on the planet where “production massively outpaces local demand, and battery technology is far from advanced enough to make it worthwhile to store and transport energy from these rural regions into the urban centres that need it” (Carter, 2021a). Icelandic geothermal power, Venezuelan subsidies, and, most importantly, Chinese enormous quantities of renewable hydro energy (especially in the regions of Sichuan and Yunnan) are wasted every year.
The CCAF, however, invites to exercise caution when suggesting such arguments. A significant majority of miners (76%), indeed, seem to use renewable energies as part of their energy mix. “However, the share of renewables in hashers’ total energy consumption remains at 39%” (Blandin et al., 2020, p. 27). Also, “unlike the power demand of Bitcoin mining machines, which is consistent all year long, the production of hydropower is subject to seasonality” (de Vries, 2019). Besides, “while it is true that the Chinese government’s strategy to ensure energy self-sufficiency has led to the development of massive hydropower capacity, the same strategy has driven public investments in the construction of large-scale coal mines”. (Blandin et al., 2020, p. 27). Maybe in the long run, indeed, crypto miners will naturally tend to establish near renewable sources of energy in their endless quest for efficiency (Stoll, 2019), but this scenario does not come up as immediate.
Pipe-to-crypto (Carter, 2021b). Another promising avenue for carbon-neutral mining is flared natural gas. The process of oil extraction today releases a significant amount of natural gas (especially methane) as a by-product: this clearly represents an opportunity for miners to employ this otherwise wasted resource.[3] It must be said that this is still a minor player in today’s arena, but first studies suggest that there is enough flared natural gas in the US alone to run almost the entire BTC network.[4]
Clearly, this monetization would still create emissions, and some argued that the practice even incentivizes the fossil fuel industry to invest more in oil (de Vries, 2021) whereas this gas could be better spent elsewhere. Nonetheless, we can acknowledge that “given the reality that oil is and will continue to be extracted for the foreseeable future, exploiting a natural by-product of the process (and potentially even reducing its environmental impact) is a net positive” (Carter, 2021b).
The ‘long run’ argument (Carter, 2021a). Miners are unlikely to continue expanding their mining operations at the current rates indefinitely. Indeed, “most of the energy is used to mine Bitcoins, not to support transactions. So unless the price of Bitcoin doubles every four years in perpetuity (which economics suggests is essentially impossible for any currency), the share of miner revenue will eventually decay to zero. And if profit margins fall, the financial incentive to invest in mining will naturally decrease.” The main counterargument to Carter’s above quoted proposition is that, from an economist perspective, BTC cannot be seen (and does not behave) as a currency. Therefore, the faith in this ‘long run’ argument may be – in the ‘long run’ – misplaced.
Proof of stake (PoS). This is currently the most explored alternative to PoW systems. In this instance, mining can only be done by people who already own a lot of the cryptocurrency. This has the potential to massively cut down resource use, simply by limiting competition. “Bitcoin enthusiasts might not like the fact that such a system is more centralized”, Professor Noah Smith comments, “but if the technology of decentralized trust is inherently expensive, libertarian ideals might have to give way to cold, hard economic necessity” (Smith, 2021).
Not everyone agrees to that, however. According to Carter (2021b), for example, ‘Proof of Stake’ is “just a fancy phrase meaning those who have the most wealth wield political control. That sounds a lot like our current system, which Bitcoin is specifically designed to solve. Bitcoin explicitly rejects politics, and doesn’t grant any special privileges based on coins held”. Moreover, “miners, who have a large influence on the development of Bitcoin, are not interested in removing the algorithm, which is central to their own business. Therefore, it is likely that Bitcoin will remain the largest energy consumer among public blockchain systems and will continue to consume a considerable amount of energy” (Stoll et al, 2021). Both counterarguments hold indeed some truth in them, but they can nonetheless be defused on two grounds: first, PoS – albeit still in its infancy – can be already set up in many different ways, such that not all of them imply a shift of control in the hands of few wealthy individuals or entities; secondly, the sheer fact that the second most-used blockchain system after Bitcoin – namely, Ethereum (ETH) – is switching to PoS [5] proves that there is indeed an actual interest into this new mechanism, and many more cryptocurrencies may follow its lead.
Private systems (Hammon et al., 2021) and off-chain transactions (de Vries, 2019). The argument proposed by Hammon and de Vries is similar. The common idea is that the financially onerous and energy-intensive nature of Proof of Work is driven by the need for security in an open system that anyone can join. Therefore, PoW is not needed where systems admit only trusted participants, with (typically) a controlling entity. Such systems can therefore use far simpler confirmation processes than Proof of Work, potentially a straightforward consensus from the majority of participants, or even a unilateral transaction confirmation by the central body. The very existence of this type of transaction within the Bitcoin ecosystem is counter-intuitive, but this could help – in certain fields – in the reduction of the environmental footprint per transaction.
[1] This is valid for any other cryptocurrency that were mined with this system.
[2] “As implied by the name, ASIC chips are hardwired to perform one type of calculation only (unlike FPGAs which can be reprogrammed to mine anything). This ensures that all resources are optimized for the task of generating hashes” (de Vries, 2021b).
[3] Depending on the regulatory framework, natural gas is either vented or flared at rig locations. Because these oil wells are frequently remote, off-grid, with no pipeline infrastructure, and unviable economics for capturing (due to low prices of natural gas), oil rig operators often combust waste gas on site. When this gas is put into a generator and used to mine Bitcoin, operators can ensure a full burn (eliminating vented methane from inefficient flaring) and can additionally mitigate the emissions produced (Carter, 2021b).
[4] According to the Energy Information Administration, 538 billion cubic feet of natural gas were vented and flared.
[5] Rumour has it, the switch will happen before the end of 2021.